A summer dilemma worthy of Solomon: how to stay cool in days of high heat and humidity without turning to traditional air conditioning, which consumes vast amounts of electricity and emits potent climate-changing greenhouse gases.
The answer potentially involves a new class of solid-state refrigerants that could enable energy-efficient and emission-free cooling. And now researchers from the Department of Chemistry and Chemical Biology have developed an environmentally friendly mechanism to enable solid-state cooling with two-dimensional perovskites. Their findings are described in a new study in Nature Communications.
“Shifting away from the vapor compression systems that have been in use for a really long time is a crucial part of the overall push toward a more sustainable future,” said Jarad Mason, the paper’s senior author and assistant professor of chemistry and chemical biology. “Our focus is looking deeply at the intrinsic properties of these materials to see what is possible in terms of solid-state cooling as a sustainable alternative.”
Also known as barocaloric materials, the two-dimensional perovskites release and absorb heat in response to pressure changes as they expand and contract. The effect is based on a phenomenon you may be familiar with if you’ve ever stretched a balloon and felt it warm up against your lips. Similarly, these materials release heat when pressurized or stressed. Without releasing any harmful emissions, this mechanism can remove heat in the solid state using low driving pressures.
The work was led by members of Mason’s lab, including Jinyoung Seo, Ryan D. McGillicuddy, Adam H. Slavney, Selena Zhang ’22, Rahil Ukani, and Shao-Liang Zheng, director of the X-ray Laboratory. Advanced tests were also performed in collaboration with scientists at the Argonne National Laboratory in Lemont, Illinois.
This new mechanism for solid-state cooling has the potential to overcome the limitations of traditional vapor-compression cooling technology, which has remained largely unchanged since the early 20th century.
Any kind of refrigeration system runs in a cycle from a low-entropy state when a material can absorb heat, thereby cooling a space, to a high-entropy state when that energy can be released in a heat sink, where it dissipates. Vapor-compression air conditioners circulate a volatile fluid refrigerant that evaporates and condenses under varying pressure through metal coils to cool an enclosed space and eject heat outside. Running vapor-compression cycles is energy-intensive, responsible currently for almost 20 percent of electricity use in buildings around the world. In addition, leaking refrigerants are more than 1,000 times more potent greenhouse gases than carbon dioxide.
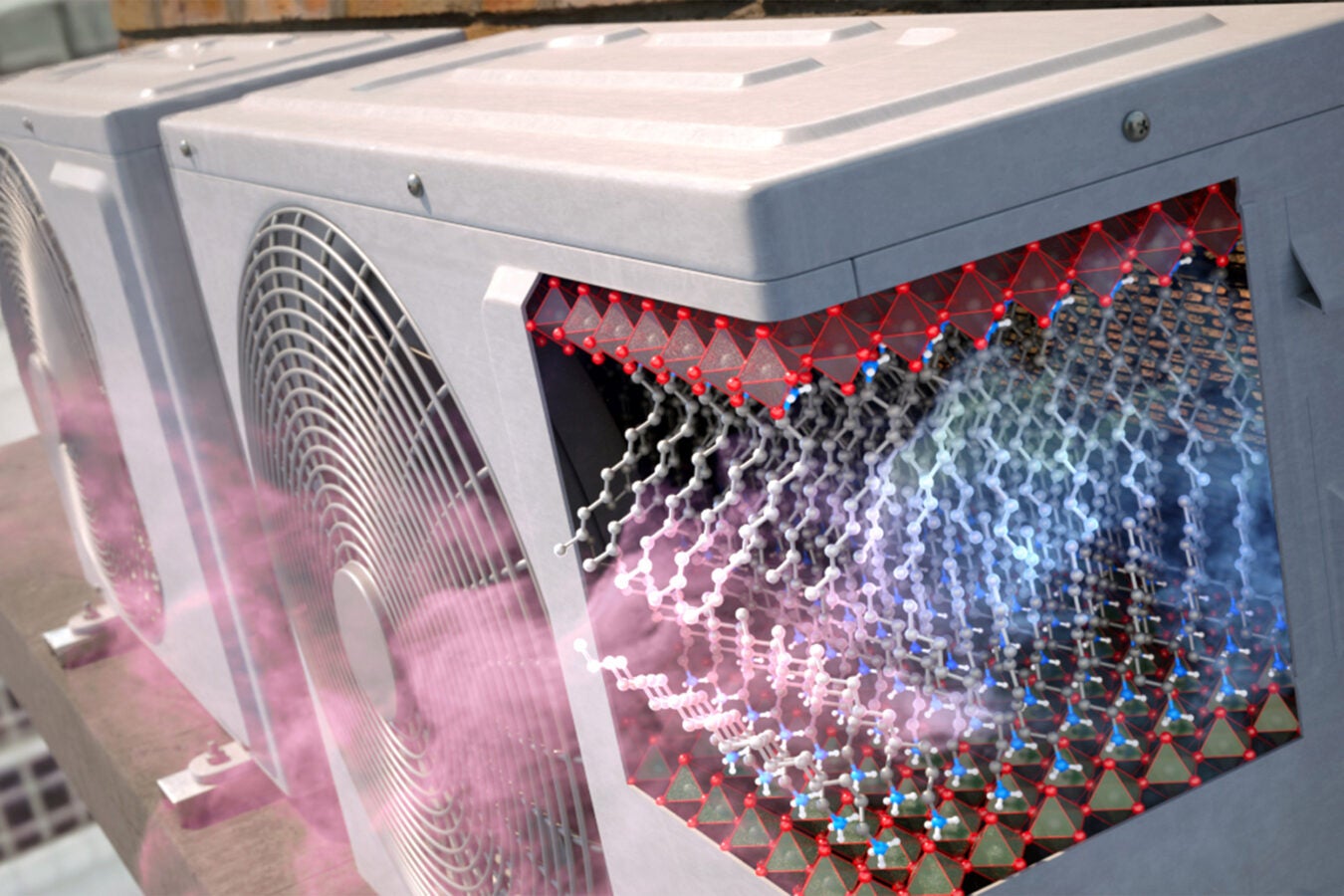
The new mechanism (pictured) could replace traditional vapor-compression cooling technology, which has remained largely unchanged since the early 20th century.
The team identified two-dimensional perovskites as ideal substitutes because they undergo phase transitions that can be driven reversibly under minimal pressure, all while remaining in a solid state; the more a material can change its entropy, the more effective it can be for running cooling cycles. With organic bi-layers capable of undergoing large changes in entropy when their hydrocarbon chains switch between ordered and disordered states, the team anticipated that two-dimensional perovskites could serve as a highly tunable solid-state cooling material that could operate at lower pressures than thought possible.
The team synthesized the materials in their lab and tested them in a high-pressure calorimeter to measure changes in heat flow in the material under varying pressures and temperatures. These experiments reveal how much heat can be removed in a potential refrigeration cycle, and how much pressure is needed to drive the cycle reversibly.
“As soon as we began testing the material, we realized that we could remove a very large amount of heat with a very small pressure change,” Mason said. “From that point on, we knew that there was going to be something interesting here.”
The researchers also conducted high-pressure powder X-ray diffraction experiments at Argonne to understand phase changes at the molecular level. With the X-ray synchrotron, the teams were able to characterize how the structures of each material changes at varying temperatures and pressures.
“These materials are worth studying beyond their promising performance,” Seo said, “They can also be useful for chemists to understand the fundamental properties that are critical to realizing this technology at scale.”
The Mason Lab next plans to craft prototype barocaloric cooling devices while continuing to explore the potential use of different materials.
“We will likely use next-generation materials for the prototype device,” Seo said. “We are trying to come up with new technologies to address the cooling challenge.”
Post a Comment